Long considered loners, many marsupials may have complex social lives
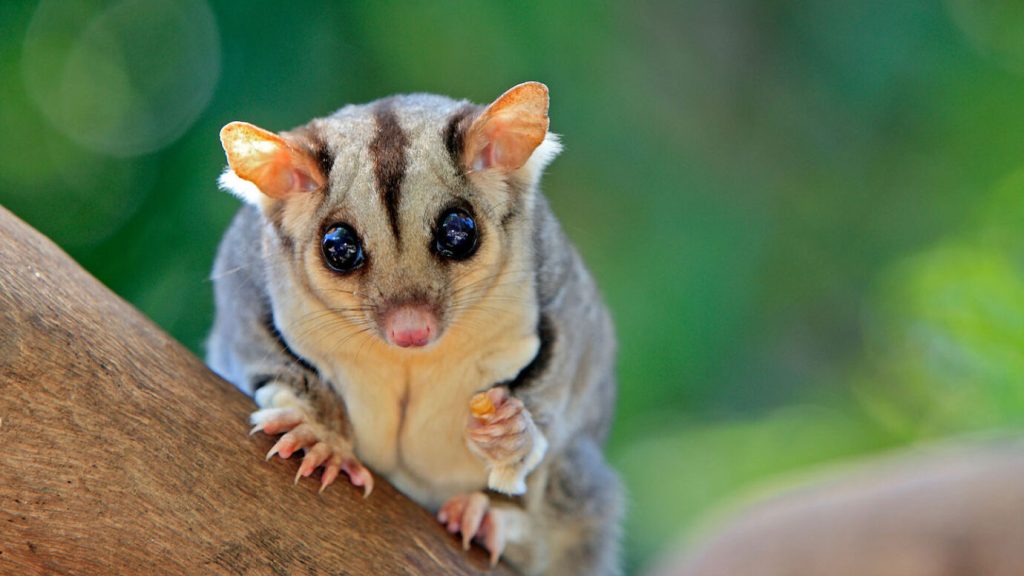
Marsupials may have richer social lives than previously thought.
Generally considered loners, the pouched animals have a wide diversity of social relationships that have gone unrecognized, a new analysis published October 26 in Proceedings of the Royal Society B suggests. The findings could have implications for how scientists think about the lifestyles of early mammals.
“These findings are helpful to move us away from a linear thinking that used to exist in some parts of evolutionary theory, that species develop from supposedly simple into more complex forms,” says Dieter Lukas, an evolutionary ecologist at the Max Planck Institute for Evolutionary Anthropology in Leipzig, Germany, who was not involved with the study.
Mammals run the gamut of social organization systems, ranging from loose, ephemeral interactions like aggregations of jaguars in the South American wetlands to the antlike subterranean societies of naked mole-rats (SN: 10/13/21; SN: 10/20/20).
But marsupials — a subgroup of mammals that give birth to relatively underdeveloped young reared in pouches — have traditionally been considered largely solitary. Some kangaroo species were known to form transient or permanent groups of dozens of individuals. But among marsupials, long-term bonds between males and females were thought rare and there were no known examples of group members cooperating to raise young. Previous work on patterns of mammalian social evolution regarded about 90 percent of examined marsupial species to be solitary.
“If you look at other [studies] about some specific species, you will see [the researchers] tend to assume that the marsupials are solitary,” says Jingyu Qiu, a behavioral ecologist at CNRS in Strasbourg, France.
Sorting social lives
Qiu and her colleagues developed a database of field studies that illuminated marsupial social organization, taking into account how populations vary within a species and delving into the evolutionary history of marsupial social lives. The researchers compiled data from 120 studies on 149 populations of 65 marsupial species, categorizing each population as solitary, living in pairs — such as one male and one female — or falling into four kinds of group living, including one male and multiple females (or vice versa), multiple males and females, or single sex groups.
While 19 species, or 31 percent of those studied, appear to go strictly solo, nearly half of the species always live in pairs or groups. The team also found lots of variation within species; 27 of the 65 species — more than 40 percent — fell into multiple social organization classifications.
When the researchers looked at this social variation against climatic conditions in Australia, they found that social variability was more common in drier environments with less predictable rainfall. It’s possible that being able to switch between solitary and group living acts as a buffer against resource unpredictability.
The researchers’ focus on social flexibility “highlights that there is nothing simple even about a supposedly solitary species,” Lukas says.
Implications for the earliest mammals
Qiu and her colleagues also ran computer analyses comparing the evolutionary relationships of the marsupials with how they form social relationships. This let the team predict the social organization of the earliest marsupials, which split from placental mammals about 160 million years ago. Because modern marsupials have been considered solitary, the marsupial ancestors — and the earliest mammals on the whole — have generally been assumed to be solitary as well.
The team found that solitary was the most likely social category of the ancestral marsupials, a 35 percent probability. But Qiu points out that the varied combinations where pair and group living are possible options make up the other 65 percent. So “it is more likely that the ancestor was also non-solitary,” she says. The findings also give insights into the range of possible lifestyles experienced by the earliest mammals, she says.
But Robert Voss, a mammalogist at the American Museum of Natural History in New York City, questions the analyses’ insights about a potentially social ancestral marsupial. The uncertainty about the solitary alternative, he says, is largely due to the researchers’ benchmarks for what does and what doesn’t constitute social behavior — thresholds that Voss views as too permissive. For example, Voss disagrees with the team’s characterization of opossum social organization.
“Anecdotal observations of [members of the same species] occasionally denning together is not compelling evidence for social behavior,” says Voss. “None of the cited studies suggest that opossums are anything other than solitary.”
Future work, Qiu says, will involve gathering data on a larger subset of mammals outside of marsupials to get a clearer picture of how social traits have evolved among mammals.